Failure is a beginning of success. Negative results gave me many insights into new directions.
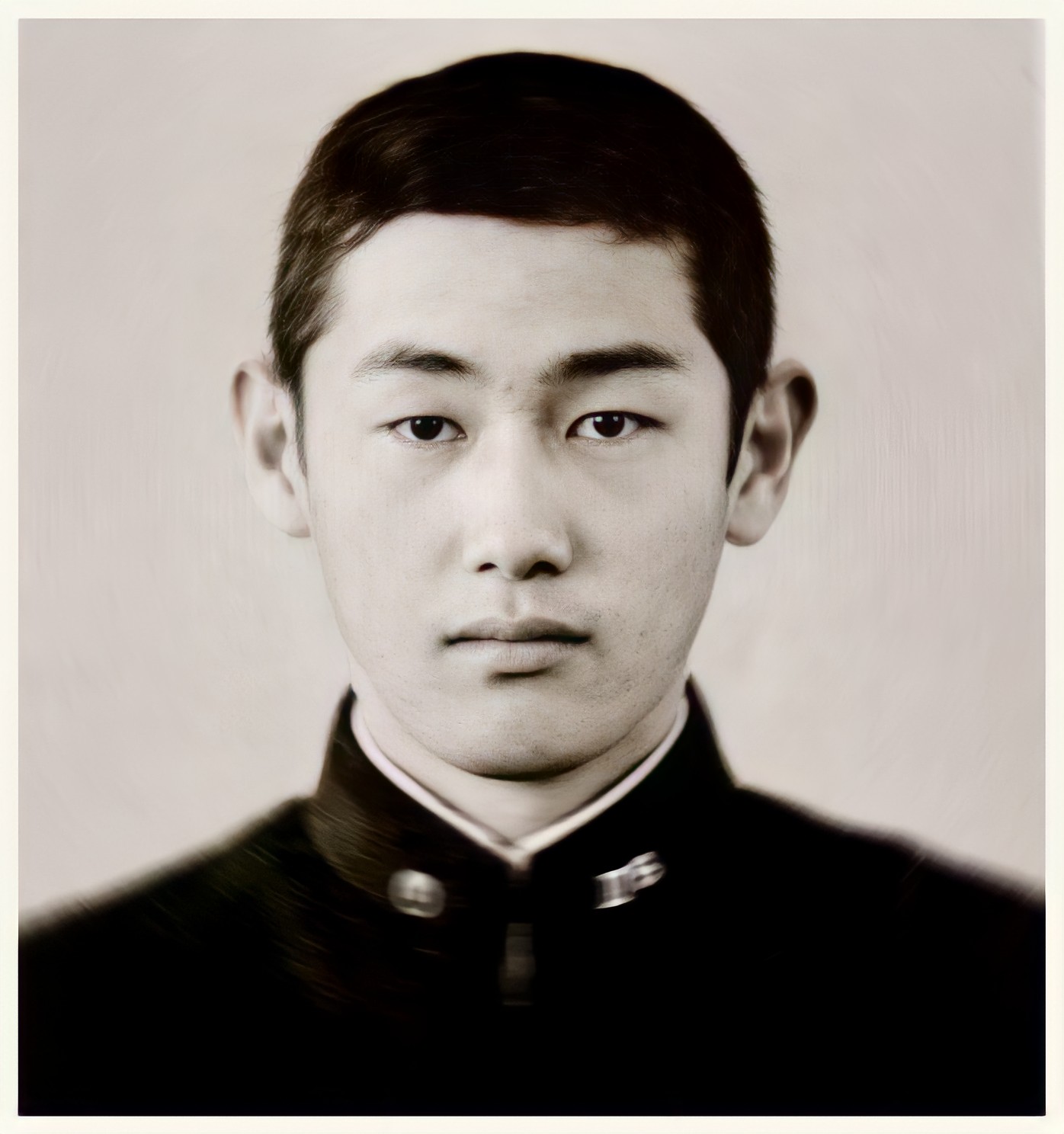
Shinya Yamanaka was born in Osaka, Japan, where his father owned a small factory. Surrounded by machinery, young Shinya developed an enthusiasm for all technical subjects. In school, he was drawn to science; he was also an enthusiastic athlete. His passion for judo resulted in a number of broken bones and frequent visits to the orthopedist. Although he ultimately earned a black belt in judo, these experiences also awakened his interest in medicine. After graduating from a high school attached to the university in Osaka, he earned a medical degree at Kobe University. He completed a residency in orthopedic surgery at National Osaka Hospital, but became frustrated with his lack of dexterity as a surgeon. He decided to pursue a career in research instead, and enrolled at Osaka University, earning a Ph.D. in pharmacology. In graduate school, a chance reading of a paper on genetically engineered mice, so-called “knockout mice,” led him to the study of gene replacement. By eliminating individual genes from the mouse genome, scientists were able to learn the function of each gene. Yamanaka was eager to work with this new technology, but there were no institutions pursuing this research in Japan. He wrote to 30 universities and laboratories in the United States, searching for a postdoctoral fellowship in the field, before finding a place at the University of California, San Francisco.
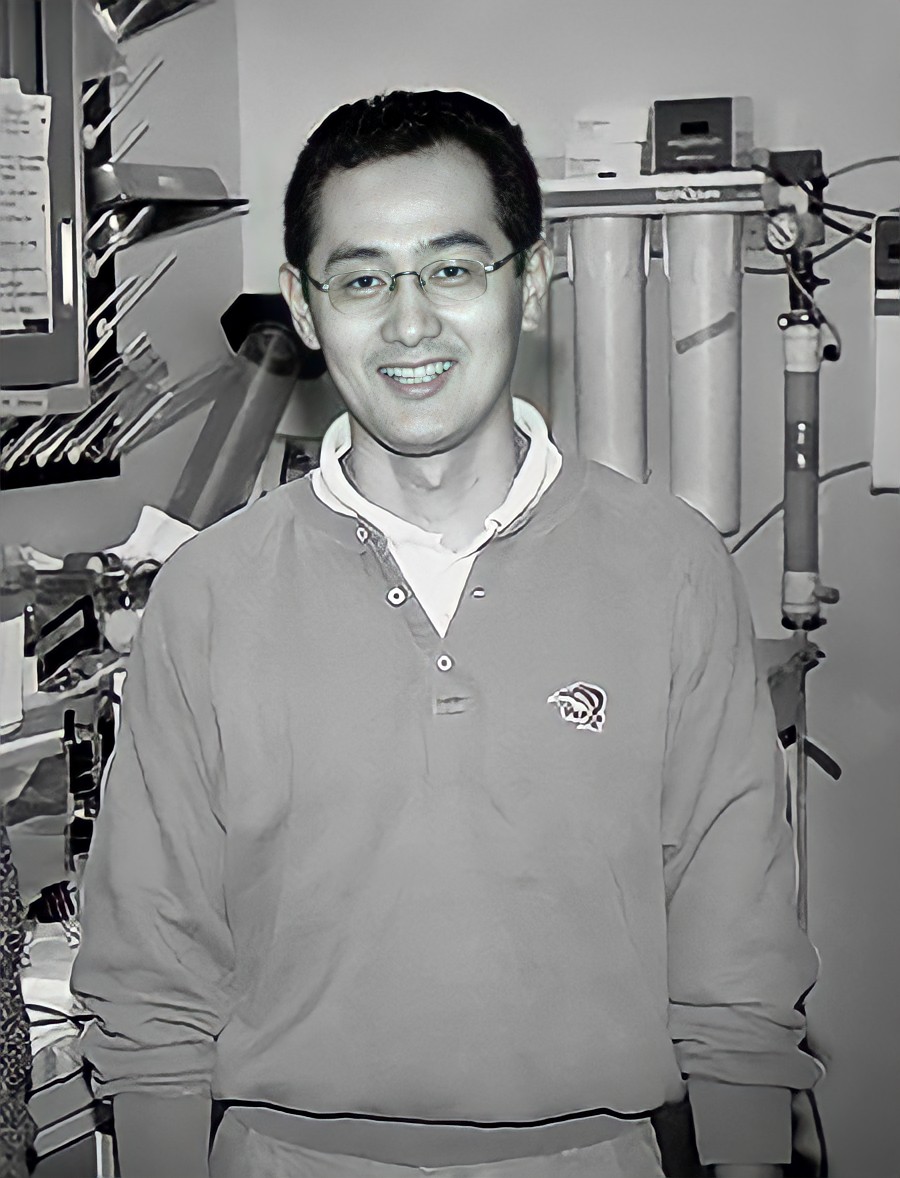
Yamanaka was thrilled with the research opportunities he enjoyed in the United States. When he was offered an assistant professorship at Osaka, he returned to Japan with a cage full of genetically engineered mice for experimentation. Unfortunately, he lacked the staff to do much more than look after his research animals, without pursuing any new projects. By this time, Yamanaka had married and started a family, but his professional life was stalled. His situation at Osaka was especially disappointing because he was eager to apply knockout mouse technology to the newly emerging field of stem cell research. Stem cells are the undifferentiated cells found in the embryos of all animals. As the embryonic creature gestates, these cells differentiate, becoming the cells that make up bone, blood, nerves and other tissues. At the close of the 20th century, stem cell research in both Japan and the United States was encumbered with a major ethical controversy. The best sources of human stem cells were the embryos discarded by fertility clinics, but destroying human embryos for research purposes struck government leaders in both countries as morally unacceptable. In 2001, U.S. President George W. Bush explicitly barred federal funding for any research involving the creation of new stem cell lines from human embryos. In Japan, the rules regarding research with embryonic tissue were even more stringent. Yamanaka considered the possibility that the cells of an adult animal could be reprogrammed to their embryonic, pluripotent state.
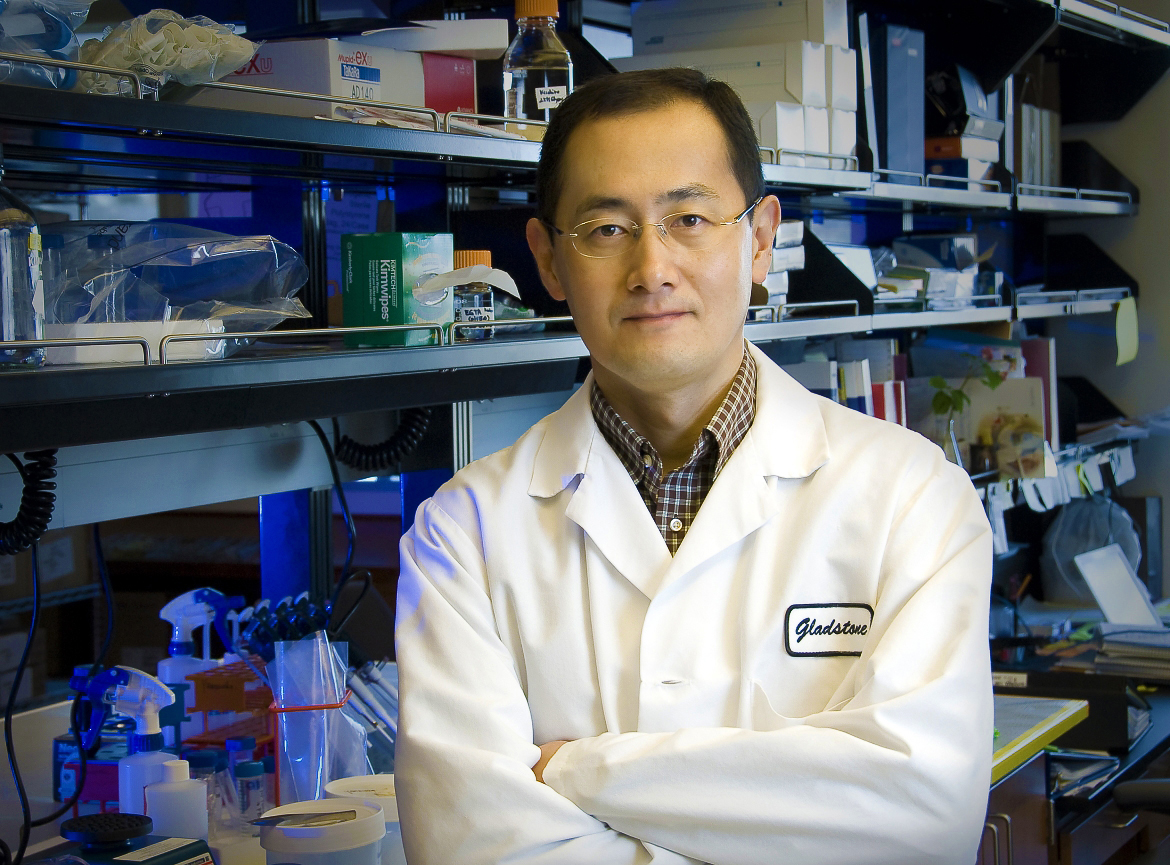
Frustrated with his lack of support at Osaka, Yamanaka considered returning to medical practice, when the opportunity arose to lead his own laboratory at another university in nearby Nara. He knew that if his new laboratory were to thrive, he would need to attract first-rate graduate students and postdoctoral fellows with a unique and intriguing research project. One day, while visiting a friend’s fertility clinic, Dr. Yamanaka found himself gazing at human embryos through a microscope and became convinced that an alternative to the use of embryonic tissue in stem cell research must be found. While most contemporary stem cell research involved controlling the process by which the stem cells differentiated into other cell types, Yamanaka decided he would pursue the opposite course, reprogramming the cells of an adult animal to return to their embryonic pluripotent state.
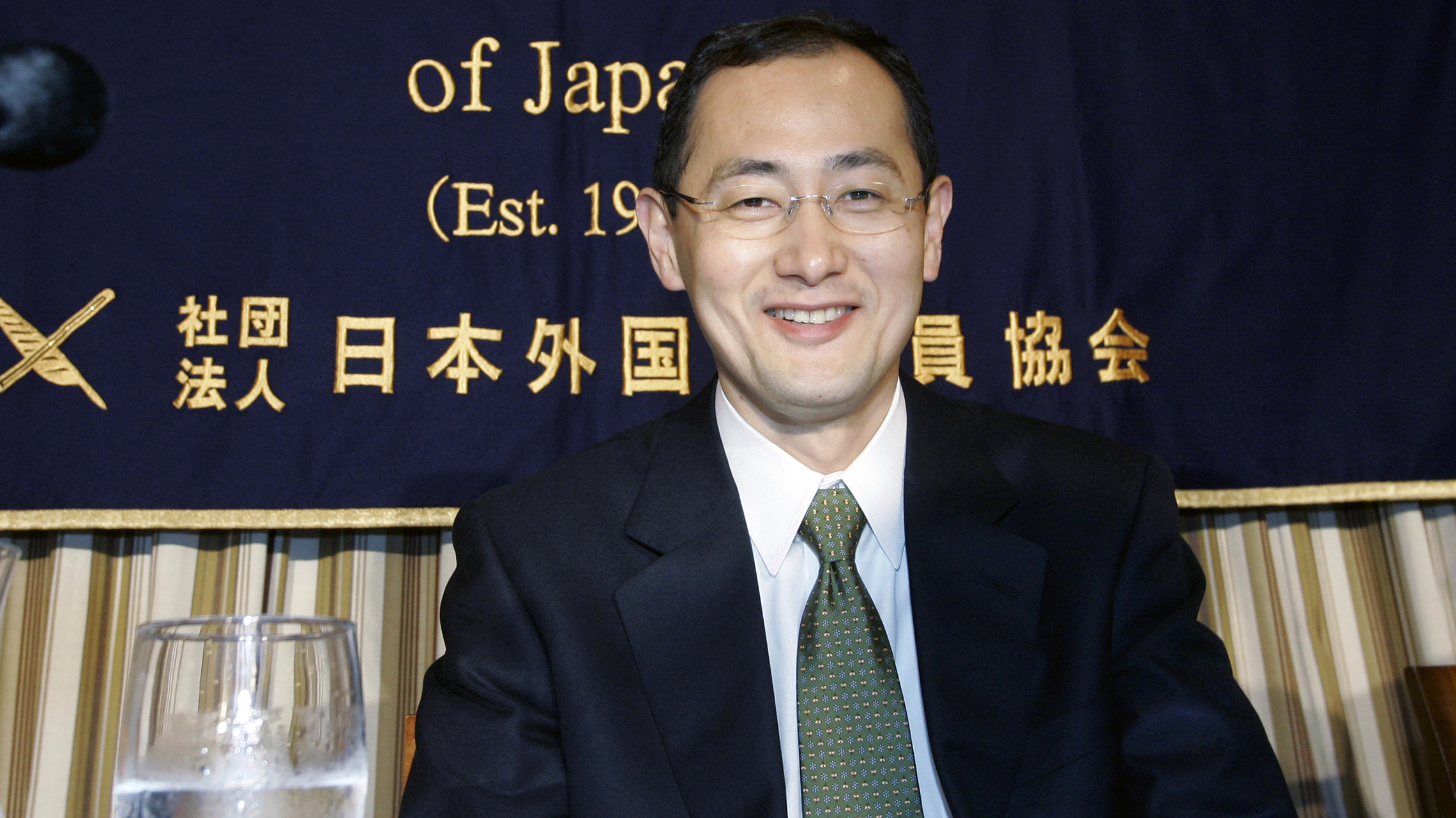
The idea seemed improbable to most of his peers, and the chances of success marginal, but the idea was so unusual, his lab attracted exceptionally talented students. Identifying the combination of genes that would convert adult cells back into their pluripotent state could require testing hundreds of possible combinations, the work of decades. Working 12 to 16 hours a day for months on end, Yamanaka reviewed the existing literature, and tried to identify the most promising combinations. Balancing meticulous research with informed intuition, he compiled a list of the 24 most likely combinations, and decided to test those first. In 2006, only five years into his project, he found a combination of four genes that converted the skin cells of an adult mouse back to their pluripotent state. His hunch had paid off, saving his team years of work.
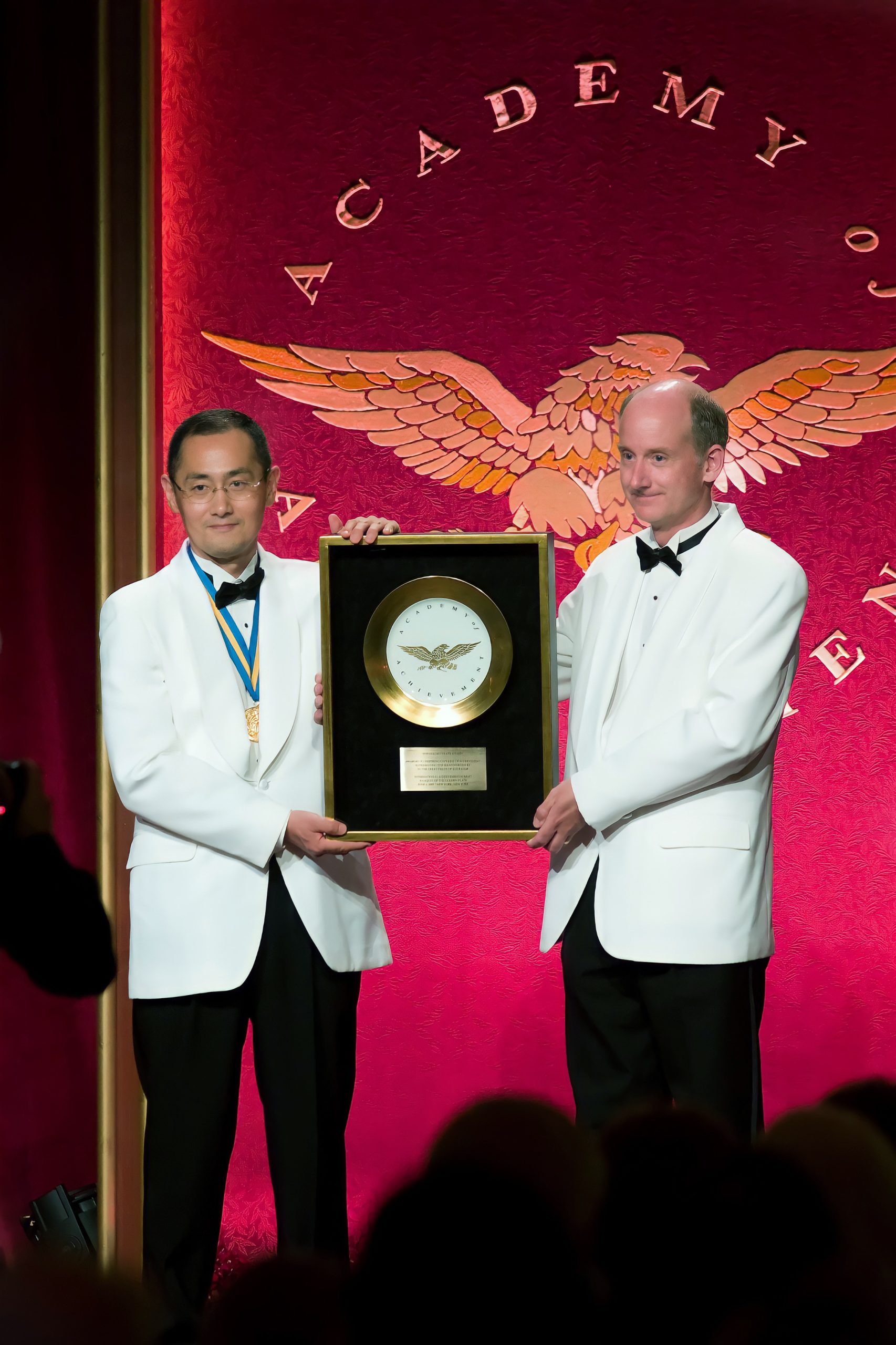
The news of Dr. Yamanaka’s discovery, quickly confirmed by other laboratories working independently, spread like wildfire through the scientific world. Everywhere, the same question sprang to mind. Would Yamanaka’s technique work with human cells as well as those of mice? An additional concern arose. Of the four gene combinations Yamanaka had used, two have been implicated in cancer. One of these has a relatively weak statistical relationship with cancer, but the other, c-Myc, is a well-known cancer gene.
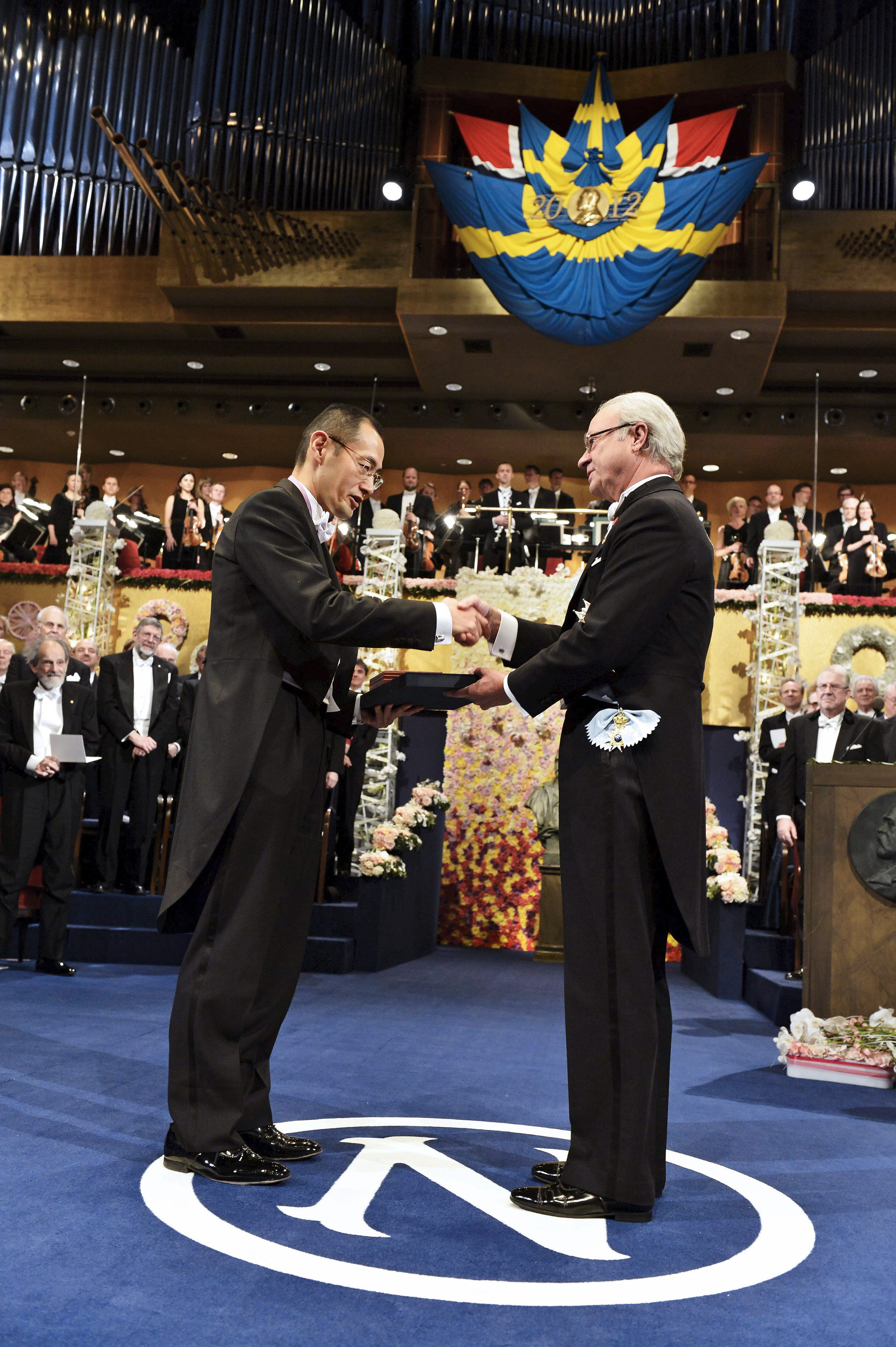
Repeating the process with human cells posed additional difficulties, including the possibility of an increased risk for cancer, but Yamanaka had become adept at overcoming difficulties and accomplishing his research goals at record speed. Within a year, Yamanaka had duplicated the results with human cells. In the process, he learned that c-Myc could be omitted from the combination, generating the same results with a greatly decreased risk of cancer. His accomplishment was recognized with honors from governments around the world, including the Osaka Science Prize in Japan, and the Lasker Award in the United States. In 2012 he received the Nobel Prize in Medicine.
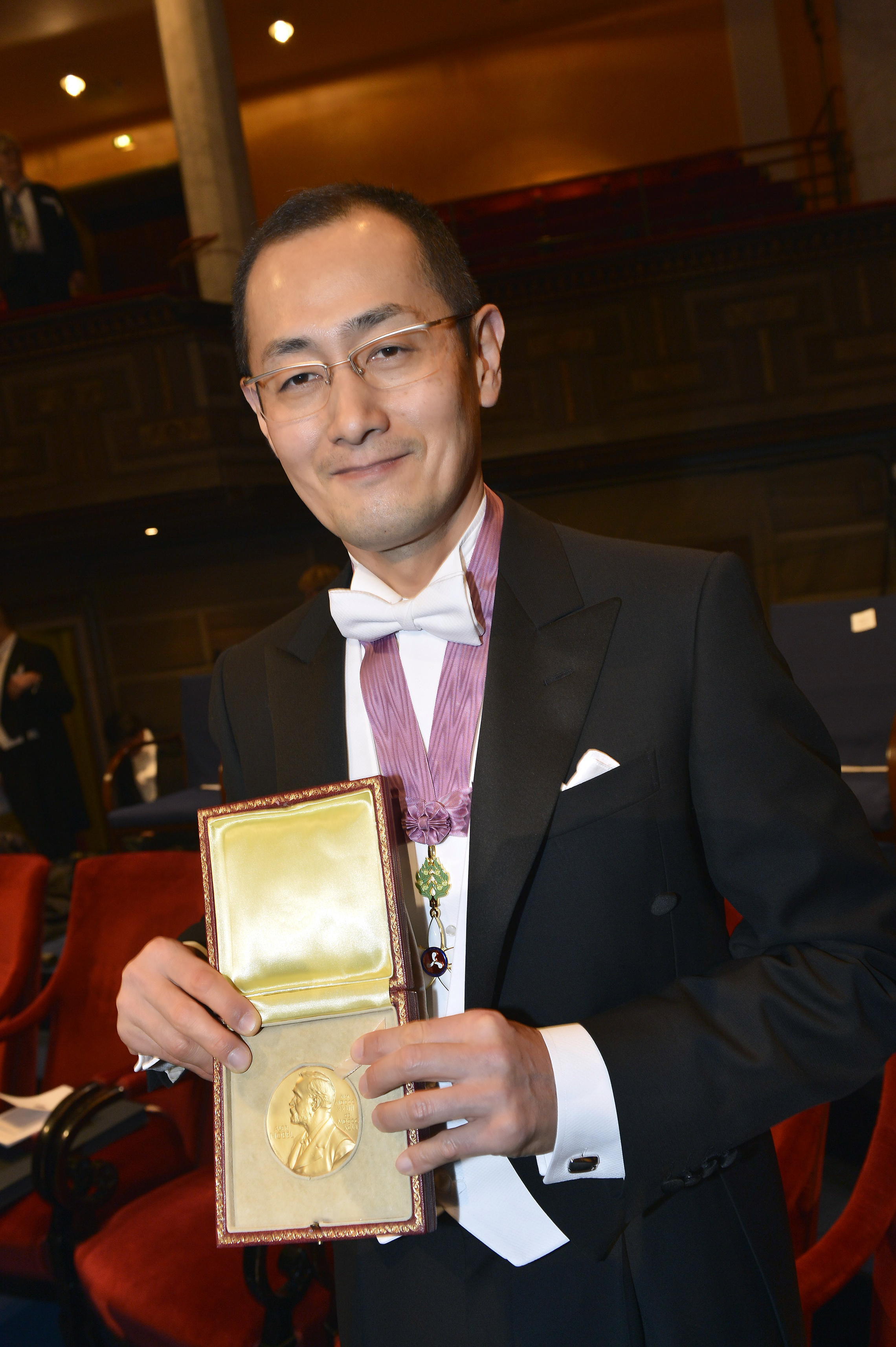
Dr. Yamanaka now divides his time between professorships at Kyoto University and the University of California’s Gladstone Institutes in San Francisco. A vigorous and athletic man, he continues to work long days, relishes his scientific pursuits and peppers his conversation with jokes in a way that his colleagues find more American than Japanese. Still an enthusiastic athlete, he enjoys a brisk run or swim between marathon sessions in the laboratory.
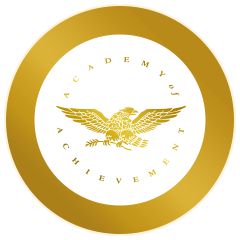
In 2007, the world of science was stunned to learn that a lone researcher, working in a small, underfunded laboratory in Kyoto, Japan had made a historic breakthrough.
For a decade, the debate over human stem cell research had pitted the ethical concerns of religious leaders and policymakers against the demands of medical research. Human stem cell research has long offered the promise of curing and preventing otherwise untreatable diseases and injuries, but the only pluripotent stem cells available for experimentation were those harvested from fetal tissue or from discarded human embryos. Moral objections to this research led a number of governments — including those of Japan and the United States — to impose stringent restrictions on further research.
When Dr. Shinya Yamanaka of the University of Kyoto succeeded in converting the skin cells of adult mice back into a stem cell-like pluripotent state, the news spread like wildfire through the scientific world. Everywhere, the same question sprang to mind. Would Yamanaka’s technique work with humans as well as mice? By the end of the year, Yamanaka had duplicated the results with human cells. His discovery was hailed by scientists and religious leaders as a breakthrough that overcame the moral objection to stem cell research.
(At the 2008 International Achievement Summit in Kailua-Kona, Hawaii, Dr. Yamanaka participated in a panel discussion of genetics in medicine. An excerpt from his remarks on that occasion precedes the text of this interview.)
Dr. Yamanaka, you’ve been exploring a new form of stem cell research. How did you become involved in this work, and what do you see as the promise of stem cell research?
Shinya Yamanaka: It may be surprising, but I was a surgeon, like 20 years ago. And I found I was terrible in the operating room. So I thought, “Well, I won’t help people by doing this!” That’s why I decided to move to basic science, and I hope it’s working. So, for the last — let me see — for the last 12 years I have been working on embryonic stem cells, ES cells. And I think probably… so please raise your hand if you know about ES cells. Oh, not all. Okay. Embryonic stem cells are stem cells which scientists generated from fertilized eggs. It was first derived from the mouse embryo in 1981, so 27 years ago. ES cells have two properties, very important properties. The first one: you can increase — you can culture — ES cells as much as you want, almost forever. The second, very important point of ES cells is that you can induce, you can make any types of cells from ES cells, including cardiac cells, neural cells, blood cells, and also germ cells. That means you can prepare any cells, in any quantity, any time. Because of that, mouse embryonic stem cells — ES cells — gave rise to a new technology called “knockout mouse” technology, which is a super technology in understanding the gene function. And as you may know, knockout mouse technology was awarded the Nobel Prize last year. Then in 1998, Dr. James Thomson developed ES cells from human blastocysts. That opened up a completely new way in regenerative medicine.
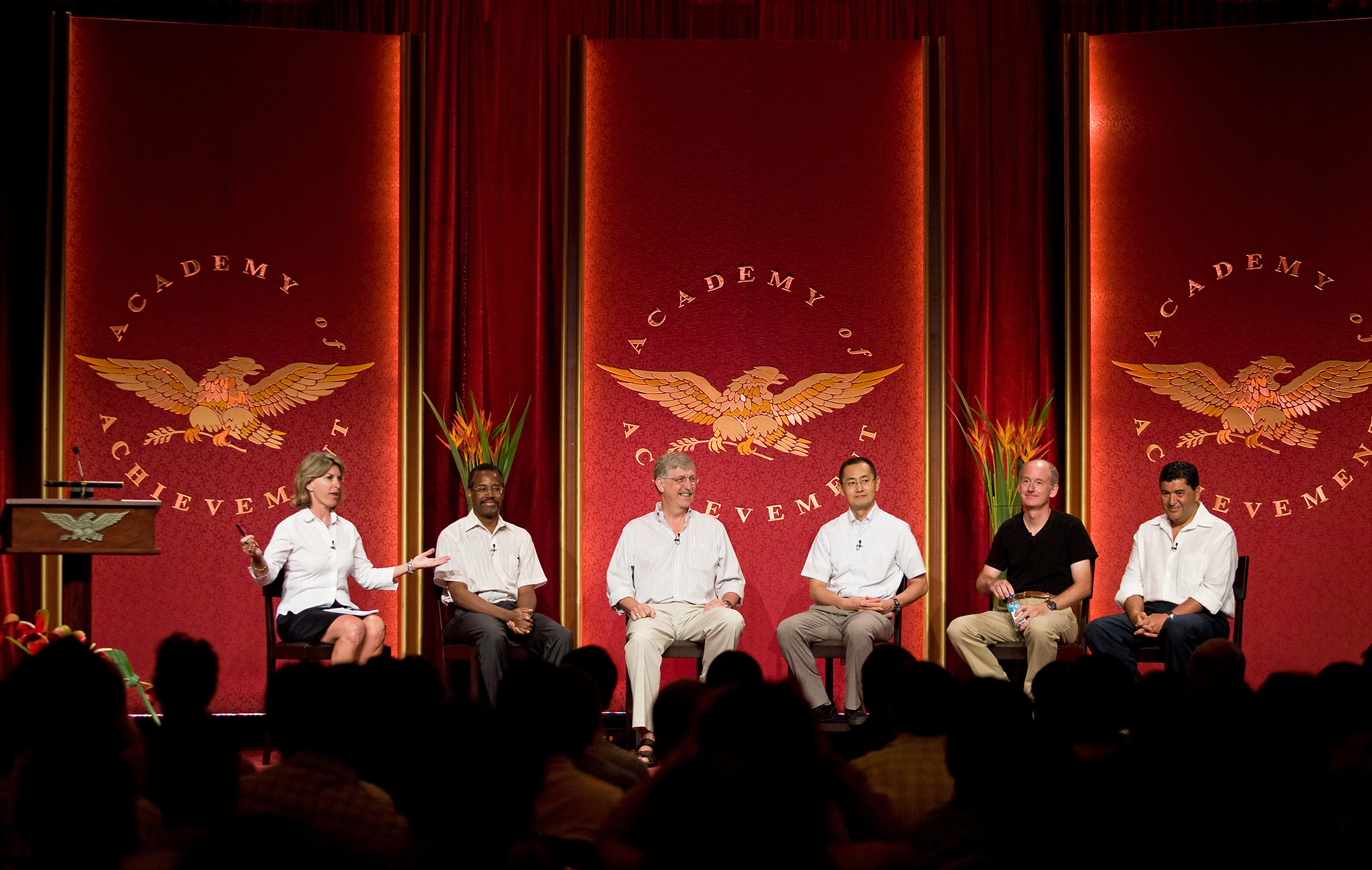
Because of his success, we have new opportunities to prepare cardiac cells and neural cells to transplant into patients. So when I saw his paper, I thought, “Wow! It’s just amazing.” But after that, I realized some problems with human ES cells.
You have to use human embryos to prepare human ES cells. And some people do not like that idea, including the president of your country. And also, because ES cells are not the patients’ own cells, we have to deal with immune rejections after transplantation. So we decided to start a new project of our laboratory, in which we tried to generate ES-like stem cells, not from embryos, but from patients’ own cells. We thought the project would be very, very risky, challenging, and it would take 20 or 30 years. But it turned out it took only five years to achieve that goal in a mouse. So we were able to publish the generation of new stem cells — which were designated “iPS cells,” induced pluripotent stem cells — from mouse skin cells in 2006. And last year we were able to translate that technology to the human. So we and James Thomson, almost at the same time, were able to report that we can make ES-like stem cells without using embryos. We can convert a patient’s skin cells directly to ES-like stem cells.
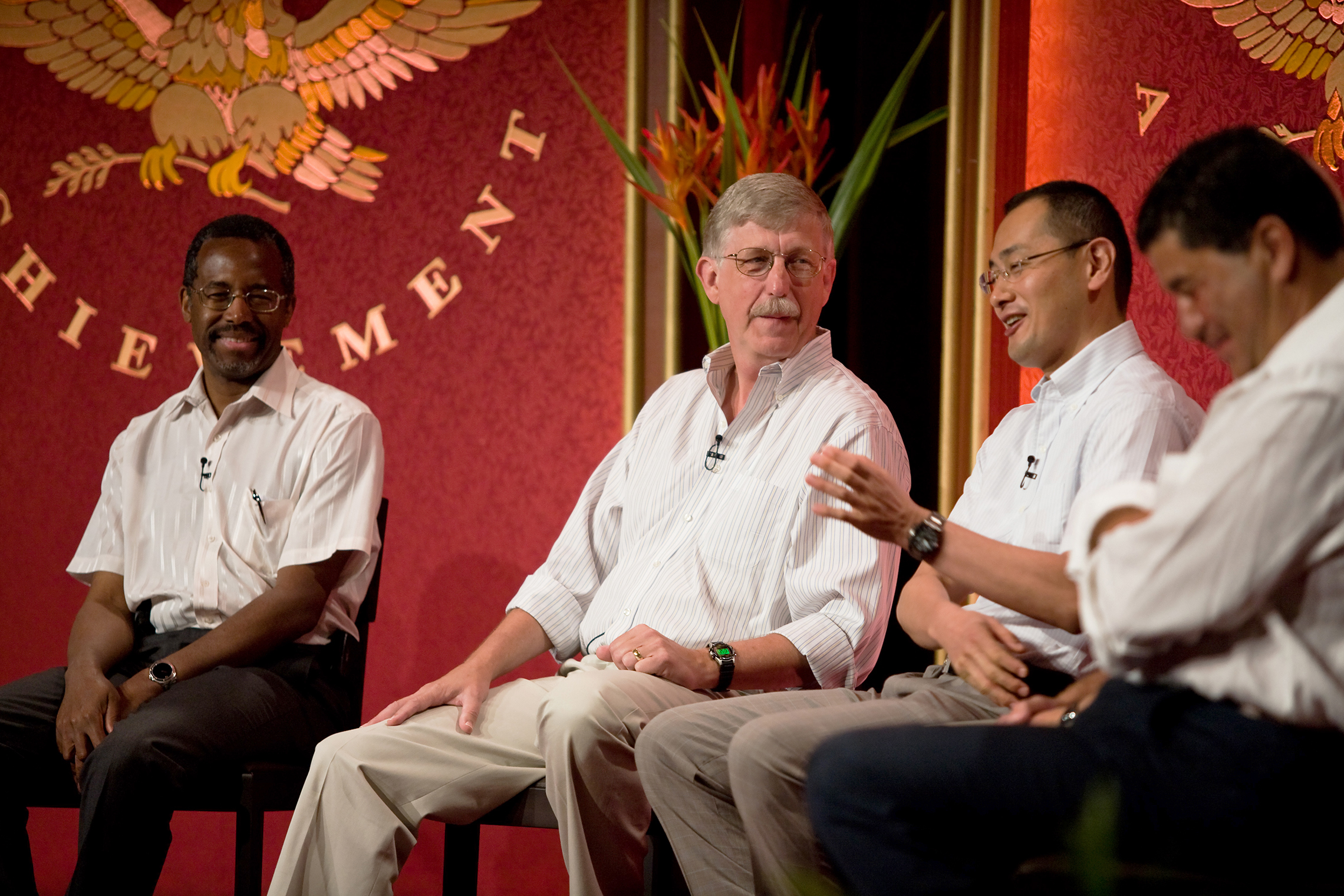
Could you clarify the significance of ES cells for us?
Shinya Yamanaka: ES cells have two important properties. The first one: we can proliferate — we can culture ES cells as much as we want. So from a single ES cell we can have a million, billion or more ES cells within a certain period of time. The other important property of ES cells is something called pluripotency. So pluripotency means that we can make any types of cells that exist in our body. We have more than 200 types of cells in our body. So we can make those 200 types of cells from ES cells. So that’s the second important property of ES cells.
Let’s take a moment to really define what your stem cell breakthrough is, and we’ll go from there to the impact it has on the future of medicine. Instead of using embryonic stem cells, you have taken skin cells, and what have you done with them?
Shinya Yamanaka: Because of this technology, iPS technology, now we can prepare many types of human cells. For example, heart cells — cardiac cells — or neural cells from patients. So you can easily imagine that without this technology, it’s impossible to take cardiac cells from patients who have some kinds of cardiac diseases. If the patient dies, we may be able to get a small amount of cardiac cells from that patient, but those cells do not proliferate, so we cannot increase the number. But with this technology, all we need is a small piece of skin from that patient, and by making iPS cells, we can increase the number of cells as much as we want. And then we can make cardiac cells from those iPS cells. So I think for the first time in the history of medicine, we now have an opportunity to prepare many, many cardiac or neural cells directly from patients, and those cells should be very, very useful, to understand why those patients become sick. And to search for very effective drugs for that patient, and also to study — to predict — any side effect for that particular patient. So that is the most beautiful approach — I mean application — of this iPS cell technology.
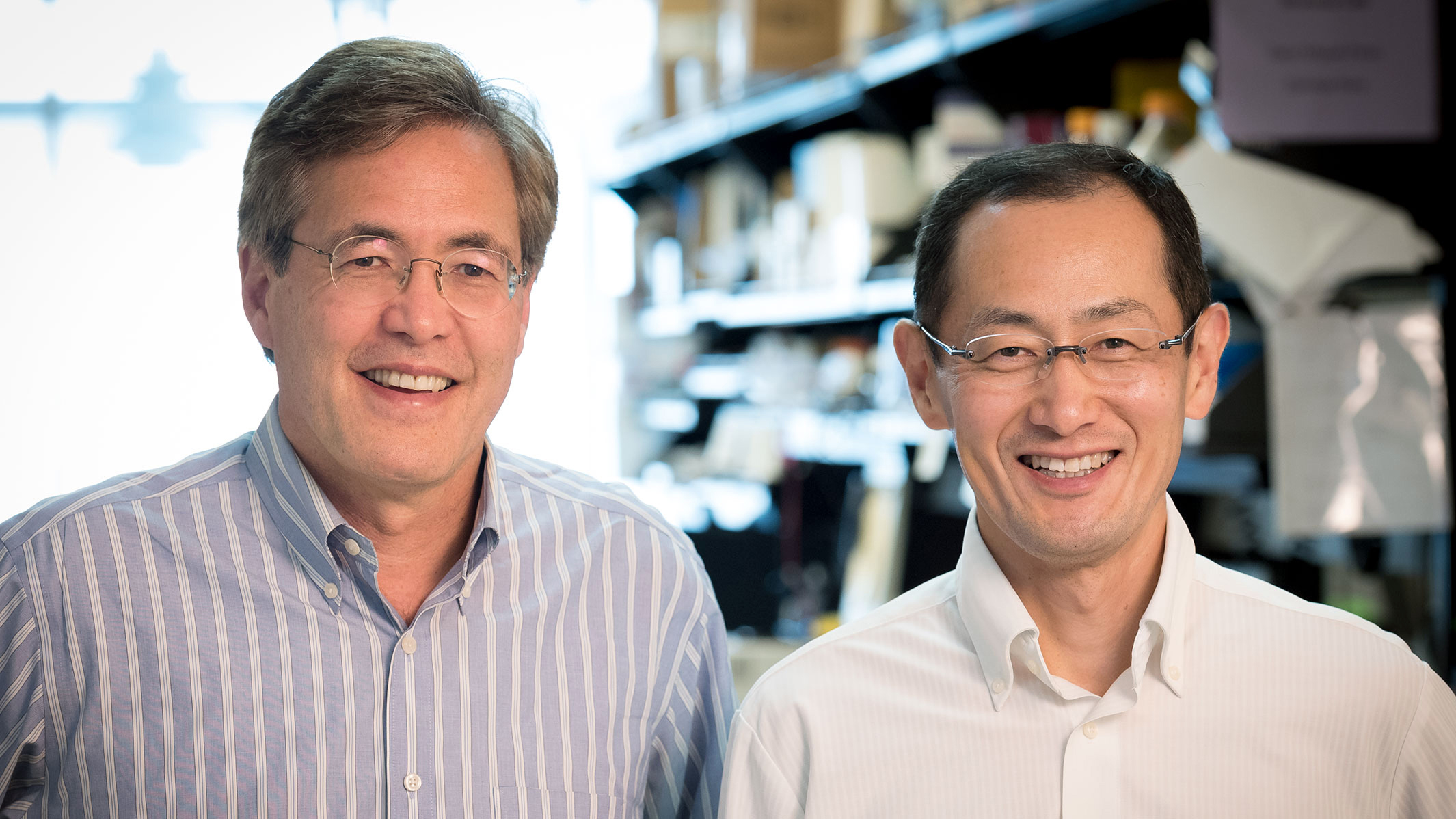
Dr. Yamanaka, many of the scientists we’ve spoken with say that failure is the biggest teacher. Have you experienced that in your career?
Shinya Yamanaka: I was an orthopedic surgeon, and my first failure was that I was not good at doing surgery, and that failure gave me an opportunity to move to basic science. Then my first major was pharmacology, and in pharmacology we only use many inhibitors and stimulators, all just drugs. And any drug cannot be 100 percent specific and 100 percent effective. So although I did many, many experiments, I did not obtain the answer, because the drugs I used weren’t specific enough. So that was kind of my second failure in my career. But that second failure got me interested in knockout mice, mouse technology. So I think failure is important in my career.
In science, because it’s based on experimentation, it seems that scientists rely on failure, not only to tell them what not to do, but what to do first.
Shinya Yamanaka: I agree, yes.
Did you start working with stem cells in graduate school?
Shinya Yamanaka: In my Ph.D. school, I didn’t work on stem cells. I just worked on basic pharmacology. But by doing pharmacology, I got very interested in so-called “knockout mouse” technology. Knockout mouse is a way to study the function of genes. You know, both human and mouse have approximately 20,000 genes. With knockout mouse technology, we can select one gene out of those 20,000 genes, and completely destroy that one particular gene so that we can understand the function of that particular gene. I got very interested in that technology. It was 1992 or 1993 when I graduated from my Ph.D. school. So I decided to study — I decided to learn about knockout mouse technology. But at that time in Japan, only a few scientists were working on knockout mouse technology. That’s why I decided to move to the States, San Francisco.